The cervical spine (Fig. 25-1), which consists of 37 joints, permits more motion than any other region of the spine. The majority of the anatomy of the cervical spine can be explained by reference to the functions that the head and neck perform on a daily basis. The head has to be able to perform extensive, detailed, and, at times, very quick motions during everyday tasks. These motions allow for precise positioning of the eyes and the ability to respond to a host of postural changes that result from stimulation of the vestibular system (see Chapter 3).1 In addition to providing this amount of mobility, the cervical spine has to afford some protection to several vital structures, including the spinal cord and the vertebral and carotid arteries. However, with stability being sacrificed for mobility, the cervical spine is rendered more vulnerable to both direct and indirect trauma.2 FIGURE 25-1 Cervical vertebra. Neck and upper extremity pain are common in the general population. Almost everyone experiences neck pain at some point in their life,3 and approximately 54% of individuals have experienced neck pain within the last 6 months.4 In the younger population, cervical pathology is most commonly due to a ligament sprain or muscle strain, whereas in the elderly cervical injuries are more commonly due to cervical spondylosis and/or spinal stenosis. Neck pain, which is characterized by episodes of exacerbation and recovery, has a large financial impact on a number of industrial countries.5 Neck pain may also coexist with signs and symptoms of distal involvement, including pain radiating down the arms and weakness or numbness in the upper extremity.6 Most individuals with cervical dysfunction are labeled as having nonspecific neck pain caused by a neuromuscular or articular dysfunction.7 Anatomically and biomechanically, the cervical spine can be divided into three regions: craniovertebral (the upper two vertebrae), the cervical spine proper (vertebrae 3–6), and the cervicothoracic junction (the seventh cervical vertebra and the first thoracic vertebra). For the sake of simplicity, these units are described separately. The craniovertebral region is described in Chapter 23, while the remaining cervical vertebrae are described in this chapter.
CHAPTER 25
The Cervical Spine
OVERVIEW
ANATOMY
Vertebrae
Compared with the rest of the spine, the vertebral bodies of the cervical spine are small and consist predominantly of trabecular (cancellous) bone.1The third to sixth cervical vertebrae can be considered typical, whereas the seventh is atypical. The third, fourth, and fifth vertebrae are almost identical, whereas the sixth has enough minor differences to distinguish it from the others.
The typical cervical vertebra has a larger transverse than anteroposterior dimension. The superior aspect of the centrum is concave transversely and convex anteroposteriorly, forming a sellar surface that reciprocates with the inferior surface of the centrum, superior to it.1 The superior surface of the vertebral body is characterized by superiorly projecting processes on their superolateral aspects. Each of these hook-shaped processes is called an uncinate process and is composed of the raised lip of the superolateral aspect of the body, which articulates with a reciprocally curved surface at the synovial uncovertebral joint.
The transverse processes of vertebrae C3–6 are posterior and lateral to the transverse foramina, through which the vertebral artery, accessory vertebral vein, and the vertebral nerve all pass. Notable structural changes occur in the lower cervical vertebrae and include spinous processes that are more elongated, point inferiorly, and lose the characteristic bifid appearance of the cervical spine. The transverse processes are short and project anterolaterally and slightly inferiorly. The costotransverse and costovertebral articulations are found in the inferior aspect of the cervicothoracic region (see Chapter 27).
With the exception of the C2 vertebra (see Chapter 23), the superior aspect of the transverse process has a deep groove that mimics the orientation of the transverse process and the spinal nerve, both of which are parallel with the intervertebral foramen. The inferolateral orientation of the transverse process and the fact that the spinal nerves are firmly anchored in the groove make the nerves vulnerable to a stretch injury around the distal end of the transverse process.1
The articular pillars and zygapophyseal (facet) joints of vertebrae C2–7 are located approximately 1 inch lateral to the spinous processes. The articular pillar is formed by the superior and inferior articular processes of the zygapophyseal joint, which bulge laterally at the pedicle–lamina junction. The articular facets on the superior articular process are concave and face superolaterally to articulate with the reciprocally curved and orientated facet on the inferior articular process of the vertebra above. The articular pillars bear a significant proportion of axial loading.8
As in the rest of the spine, the pedicles and laminae form the neural arch, which encloses the vertebral foramen. The pedicles project backward and laterally, whereas the long, narrow laminae run posteriorly and medially, to terminate in a short bifid spinous process, which projects slightly inferiorly.1 The seventh cervical vertebra varies from the typical cervical vertebra. In addition to possessing a much longer and monoid spinous process to which the ligamentum nuchae attaches, the seventh vertebra has wider transverse processes, no inferior uncinate facet, and no transverse foramen.
Intervertebral Disk
In the cervical spine, there are five disks, with the first disk being located between C2 and C3. The cervical disks (Fig. 25-2) are named after the vertebra above (C4 disk lies between C4 and C5). The anatomy of the cervical disk is distinctly different from that of the lumbar intervertebral disk (IVD). The cervical nucleus pulposus (NP) at birth constitutes no more than 25% of the entire disk, not 50% as in lumbar disks.9
FIGURE 25-2 The intervertebral disk, articulations, ligaments, and neurologic structures. (Reproduced, with permission, from Chapter 1. Back. In: Morton DA, Foreman K, Albertine KH. eds. The Big Picture: Gross Anatomy. New York, NY: McGraw-Hill; 2011.)
The IVD height-to-vertebral body height ratio (2:5) is the greatest in the cervical spine, and the IVDs make up approximately 25% of the superior-to-inferior height of the cervical spine.10 This increase in height ratio allows for the greatest possible range of motion. The NP sits in, or near, the center of the disk, lying slightly more posteriorly than anteriorly. The inferior surface of the cervical IVD is concave, and the inferior–anterior surface of the centrum projects downward to partly cover the anterior aspect. The anterior margin of the IVD is attached to the anterior longitudinal ligament (ALL). This surface can be palpated by the clinician and is often tender in the presence of intervertebral instability. The diskal margins of the posterior surface of the vertebral body give rise to the posterior longitudinal ligament (PLL).
A number of features distinguish the cervical disk from the lumbar disk11:
Anteriorly, the cervical annulus fibrosus (AF) consists of interwoven alar fibers, whereas posteriorly, the AF lacks any oblique fibers and consists exclusively of vertically orientated fibers.
Essentially, the cervical AF has the structure of a dense anterior interosseous ligament, with few fibers to contain the NP posteriorly.
In no region of the cervical AF do successive lamellae exhibit alternating orientations. In fact, only in the anterior portion of the AF—where obliquely orientated fibers, upward and medially, interweave with one another—does a cruciate pattern occur.
Posterolaterally, the NP is contained only by the alar fibers of the PLL, under or through which the nuclear material must pass if it is to herniate. Further protection against disk herniation is afforded by the uncovertebral joints, which reinforce the posterolateral aspect of the IVD.
The absence of an AF over the uncovertebral region. In this region, collagen fibers are torn during the first 7–15 years of life, leaving clefts that progressively extend across the back of the disk. Rather than an incidental age change, this disruption has been interpreted either as enabling12 or resulting from13 rotatory movements of the cervical vertebrae (see next section).
Axial rotation of a typical cervical vertebra occurs around an oblique axis perpendicular to the plane of its facets.13
As in the rest of the spine, the cervical IVD functions as a closed but dynamic system, distributing the changes in pressure equally to all components of the container (i.e., the end plates and the AF) and across the surface of the vertebral body. It has been observed that in the first and second decades of life, before complete ossification occurs, lateral tears occur in the AF, most probably induced by the motion of the cervical spine in the bipedal posture.14 The tears in the lateral part of the disk tend to enlarge toward the medial aspect of the IVD. The development of such tears through both sides may result in a complete transverse splitting of the disk. Such a process can be observed in the second and third decades of life in the lower cervical spine when the IVD is split in the middle into equal halves.14 With this aging process, the NP rapidly undergoes fibrosis such that, by the third decade, it is fibrocartilaginous in nature.15 Almost everyone older than 40 years of age has evidence of cervical disk degeneration.16 According to Töndury and Theiler,17 in the fourth and fifth decades of life, the NP usually dries out, and acute extrusion is not expected then. This development can be visualized on conventional radiographs as a flattening of the uncovertebral processes and a narrowing of the IVD space.18 This may lead to a loss of elasticity and increased stresses on the vertebral end plates.
These age-related changes are evident chemically and morphologically and are much more evident in the NP than in the AF. Degenerative disk disease (DDD) in this region results in diminished disk height, subjecting the bony elements to increased load.10 The splitting of the IVD in the second and third decades of life may result in segmental instability.14 Also, such splitting of the IVD can allow the NP to move toward the spinal canal, ultimately causing disk protrusion or extrusion and resulting in nerve root compression.14
In general, compression of the cervical nerve roots occurs at the entrance zone of the intervertebral foramina.19 Anteriorly, compression of the nerve roots is likely caused by protruding disks and osteophytes of the uncovertebral region, whereas the superior articular process, the ligamentum flavum, and the periradicular fibrous tissues often affect the nerve posteriorly.19–22 Osteophytes develop in response to this increased stress and from degenerative changes of the zygapophyseal and uncovertebral joints. The osteophytes effectively increase the available surface area and decrease the overall force on the end plates.23 Radicular arteries within the dural root sleeves may become compressed by osteophytes, leading to spasm and decreased vascular perfusion.23 In addition, venous obstruction may occur, resulting in edema and additional reduction in nerve root perfusion.23
The nerve root is divided into the anterior (ventral) and posterior (dorsal) roots (Fig. 25-2), which are responsible for motor and sensory functions, respectively. Ebraheim et al.24 described the quantitative anatomy of the cervical nerve root groove and divided it into three zones: medial zone (pedicle), middle zone (vertebral artery foramen), and lateral zone. The medial zone of the cervical nerve root groove corresponds to the intervertebral foramen, and this zone is believed to play an important role in the etiology of cervical radiculopathy.19 The intervertebral foramen, which can be further divided into an entrance zone (medial) and an exit zone (lateral), resembles a funnel in shape. The entrance zone corresponds to the narrow part of the funnel, and the shape of the radicular sheath is conical, with its takeoff points from the central dural sac being the largest part.19 Consequently, nerve root compression occurs mostly in the entrance zone of the intervertebral foramina. Whether compression of the anterior (ventral) roots, posterior (dorsal) roots, or both occurs depends on various anatomic structures around the nerve roots. Anteriorly, compression of the nerve roots is likely caused by protruding disks and osteophytes of the uncovertebral region, whereas the superior articular process, the ligamentum flavum, and the periradicular fibrous tissues often affect the nerve posteriorly.19–22 Osteophytes may develop in response to this increased stress and form degenerative changes of the zygapophyseal and uncovertebral joints. The osteophytes effectively increase the available surface area and decrease the overall force on the end plates.23 Radicular arteries within the dural root sleeves may become compressed by osteophytes, leading to spasm and decreased vascular perfusion.23 In addition, venous obstruction may occur, resulting in edema and additional reduction in nerve root perfusion.23
Articulations
The structure of the cervical vertebrae, combined with the orientation of the zygapophyseal facets, provides very little bony stability, and the lax soft tissue restraints permit large excursions of motion.1 A narrow space exists between the spinal cord and the vertebral canal walls in this region. There is also a very small amount of extra space in the intervertebral foramina. Thus, a relatively small change in either the vertebral canal or the dimensions of the intervertebral foramen can result in significant compression of the spinal cord or the spinal nerve, respectively.25
Each pair of vertebrae in this region is connected by a number of articulations: a pair of zygapophyseal joints, two uncovertebral joints, and the IVD.
Zygapophyseal Joints
There are 14 zygapophyseal joints (Fig. 25-2) from the occiput to the first thoracic vertebra (C1–7). These joints are typical synovial joints and are covered with hyaline cartilage. The articular facets are teardrop shaped, with the superior facet facing upward and posteriorly while the inferior one faces downward and anteriorly. In the middle to lower cervical regions, the dual requirements of stability and mobility are provisioned through zygapophyseal joints, with C5–6 contributing the greatest segmental mobility. The average horizontal angle of the joint planes of the middle segments is approximately 45 degrees between the frontal and transverse planes26,27 with the upper cervical levels being closer to 35 degrees, and the lower levels at approximately 65 degrees.
The orientation of the zygapophyseal joint planes in the midcervical spine permits considerable flexibility in the motions of flexion and extension and encourages the coupling motions of rotation and side bending to the same side in the upper segments (see Chapter 22). However, the more caudal segments approaching the cervicothoracic junction show a tendency for a smaller range of motion—it is here that axial loading is higher, and the segmental mobility becomes markedly diminished as the thoracic cage commences.28
The anterior joint capsule is strong, but it is lax in neutral and extension.29 The posterior capsule is thin and weak. This laxity allows for translation between facets. The major constraints and supports of these joints are the ligaments of the vertebral column and the IVD.
Vascular, fat-filled, synovial intra-articular inclusions30 have been observed in these joints. These structures have been described as fibroadipose meniscoids, synovial folds, and capsular rims. The meniscoids consist of connective and fatty tissue that is highly vascularized and innervated.14 In the cervical spine, the meniscoids function as space fillers for the uneven articular surfaces, especially in regions where the elasticity of the relatively thin cartilage is not sufficient.14 These inclusions, which are theorized to play some role in protecting the articular surfaces as they are sucked in or expelled during movements, are prone to entrapment and can play a potential role in intra-articular fibrosis and cervical spine pain.31 Töndury and Theiler17 have observed that the meniscoids atrophy and virtually disappear with increasing age.
The zygapophyseal joint receives its nerve supply from the medial branches of the posterior cervical rami from C2 to C8 and the recurrent meningeal (sinuvertebral) nerve. The recurrent meningeal (sinuvertebral) nerve also innervates the anterior dural sac, the posterior AF, and the PLL. The capsular pattern of the zygapophyseal joint is a limitation of extension and equal loss of rotation and side bending, with flexion being unaffected.
Uncovertebral Joints
From C3 to T1, there usually are a total of 10 saddle-shaped, diarthrodial articulations. These articulations, known as joints of Luschka, uncinate processes, or uncovertebral joints, are formed between the uncinate process found on the lateral aspect of the superior surface of the inferior vertebra and the beveled inferolateral aspect of the superior vertebra.32 The uncovertebral joints develop within the first 12 years of life, as a result of loading from the head, and become fully developed by about 33 years of age.33,34 Two of these uncovertebral joints are found between each pair of adjacent vertebrae in the cervical spine proper (C2–3 to C6–7). The uncovertebral joint maintains a synovial compartment and creates the posterior lateral border of the IVD.35
The biomechanical role of the uncovertebral joint is thought to be that of a sagittally oriented guiding rail during cervical flexion and extension that acts to transfer rotation forces into side-bending and posterior translation motions.32,36,37 This function is accomplished while simultaneously ensuring that the translation and, to some extent, the side bending (lateral flexion) between adjacent vertebral bodies are limited to the sagittal plane.32,36,37 This stabilizing feature develops and changes with age and is based on alterations in the uncinate processes.38
Penning and Wilmink39 highlighted a possible correlation between uncovertebral joint configuration and the coupled cervical segmental motion of side bending and axial rotation. A more recent study of the C5–6 segment level by Clausen et al.40 found that both the zygapophyseal joints and the uncovertebral joints are the major contributors to coupled motion in the lower cervical spine and that the uncinate processes effectively reduce motion coupling and primary cervical motion (motion in the same direction as load application), especially in response to axial rotation and side-bending loads.40
The onset of degenerative changes in the cervical spine has been shown to occur more commonly at the midcervical level than at the lower cervical level.41 The reasons for the different degrees of involvement are not clear. The degenerative changes result in the substitution of a hinge-like motion, with the pivot point on the contralateral side, in place of the normal gliding motion at the uncovertebral joints.1 This alteration effectively transforms the cervical segment into a sellar joint.17,39,42
The nerve roots in the midcervical level are more predisposed to osteophytic compression because of a combination of
higher uncinate process;
the smaller anteroposterior diameter of the intervertebral foramina;
a longer course of nerve roots in close proximity to the uncovertebral joints at C4–6 levels;
greatest segmental mobility at C5 and C6.
The vertebral artery may also be compromised in the degenerative cervical spondylotic process. When involved, the vertebral artery (see Chapter 24) is usually compromised at the level of the inferior aspect of the superior vertebra, where the apical posterolateral uncovertebral osteophytes are present.
Intervertebral Foramina
The intervertebral foramina are found between all vertebrae of the spine, except in the upper cervical spine. Although there are seven cervical vertebrae, there are eight intervertebral foramina. This difference occurs because there is a nerve root existing between the occiput and the atlas (C1) that is designated to the C1 nerve root. The cervical intervertebral foramina are 4–5 mm long and 8–9 mm high. They extend obliquely anteriorly and inferiorly from the spinal canal at an angle of 45 degrees in the coronal plane.43 The anterior boundary of the foramen is formed by the IVD and by portions of both vertebral bodies, with the zygapophyseal joints serving as the posterior boundaries. The pedicles form the superior and inferior boundaries. The medial to lateral depth of the posterior wall is formed by the lateral aspect of the ligamentum flavum.
The intervertebral foramina serve as the principal routes of entry and exit for the neurovascular systems to and from the vertebral canal. Within each foramen are
a segmental mixed spinal nerve;
from two to four recurrent meningeal nerves or sinuvertebral nerves;
various spinal arteries;
plexiform venous connections.
Because they contribute to the innervation of the upper limb, the lower cervical spinal nerves are quite large in diameter and nearly fill the foramina. This region is vulnerable to narrowing with certain motions, or with osteophyte growth. The dimensions of the intervertebral foramina decrease with full extension and ipsilateral side bending of the cervical spine such that uncovertebral osteophytes may compress the nerve root and cervical cord posteriorly.
The spinal nerves also are in close proximity to both the ligamentum flavum and the zygapophyseal joint. Thus, zygapophyseal joint arthritis or a hypertrophic ligamentum flavum can cause posterior impingement of these nerves.
Vertebral Canal
In the cervical region, the vertebral canal contains the entire cervical part of the spinal cord, as well as the upper part of the first thoracic spinal cord segment. There are eight cervical spinal cord segments, and thus eight cervical spinal nerves (see Chapter 3) on each side, but only seven cervical vertebrae.
Ligaments
Both the function and the location of the ligaments in this region are similar to that of the rest of the spine. For the purposes of these descriptions, the short ligaments that interconnect the adjacent vertebrae are classified as segmental, whereas those that attach to the peripheral aspects of all of the vertebrae are classified as continuous.
Continuous Ligaments
Anterior Longitudinal Ligament. The ALL (Fig. 25-2) is a strong band, extending along the anterior surfaces of the vertebral bodies and IVDs, from the front of the sacrum to the anterior aspect of C2. The ALL is narrower in the upper cervical spine but is wider in the lower cervical spine than it is in the thoracic region. It is firmly attached to the superior and inferior end plates of the cervical vertebrae, but not to the cervical disks. At the waist of the centrum, the ligament thickens to fill in the concavity of the body. The ALL functions to restrict spinal extension and is thus vulnerable to hyperextension trauma.
Posterior Longitudinal Ligament
Lying on the anterior aspect of the vertebral canal, the PLL extends from the sacrum to the body of the axis (C2), where it is continuous with the tectorial membrane (Fig. 25-2). The PLL travels over the posterior aspect of the centrum, attaching to the superior and inferior margins of the body, but is separated from the waist of the body by a fat pad and the basivertebral veins. In addition, this ligament attaches firmly to the posterior aspect of the IVDs, laminae of hyaline cartilage, and adjacent margins of vertebral bodies. The PLL is broader and considerably thicker in the cervical region than in the thoracic and lumbar regions.44 The PLL functions to prevent disk protrusions and also acts as a restraint to segmental flexion of the vertebral column.
The dura mater is strongly adherent to the PLL at the level of C3 and higher, but this attachment diminishes at lower levels.
Ligamentum Nuchae (Nuchal Ligament)
This bilaminar fibroelastic intermuscular septum spans the entire cervical spine, extending from the external occipital protuberance to the spinous process of the seventh cervical vertebra.45 Some consider the ligament to be an extension or a replacement of the supraspinous and interspinous ligaments.46,47 However, an observational study by Allia and Gorniak48 suggests that it is a distinct ligamentous structure comprised of four portions formed by aponeurotic fibers from the trapezius, splenius capitis, rhomboid minor, and the serratus posterior superior.47 The ligament is described as being a strong, thick band of elastic tissue that aids in the suspension of the head and neck.47 However, the function of the nuchal ligament is controversial. While Mercer49 suggests that the only contribution of the ligament to stability is at C7 and the occiput, others have proposed that the ligament may play a more important role in cervical stability,48,50 while still others have proposed that it many play a role in posterior dural stretching and cervicogenic headaches. Studies47–49 have shown that when the occipitoatlantal joint is flexed, the superficial fibers of the ligament tighten and pull on the deep laminae and muscular attachments, which, in turn, pull the vertebrae posteriorly, limiting the anterior translation of flexion and, therefore, flexion itself.
Segmental Ligaments
The interspinous ligaments are thin and almost membranous, interconnecting the spinous processes. The ligament is poorly developed in the upper cervical spine but well developed in the lower.44
The ligamentum flavum runs perpendicularly to the spine from C1 and C2, where it is referred to as the posterior atlantoaxial ligament, to L5 and S1. This ligament connects the laminae of successive vertebrae, from the zygapophyseal joint to the root of the spinous process. It is formed by collagen and yellow elastic tissue and, therefore, differs from all other ligaments of the cervical spine. The ligamentum flavum of the cervical spine is fairly long, allowing an appreciable amount of flexion to occur while maintaining tension when the head and neck are in neutral. Scarring or fatty infiltration to the ligament in this region can compromise the degree of elasticity, making the ligament lax, particularly with cervical extension. This laxity increases the potential for the contents of the vertebral canal to be compressed by the buckling ligament.51 Any enlargement of the ligament increases the likelihood that a spinal nerve or its posterior root will become impinged.9 The ligament appears to function as a restrictor of flexion of the neck.
Muscles
The neck muscles are characteristically grouped in layers52:
The superficial layer consists of muscles that connect the skull and shoulder girdle and include the trapezius (Fig. 25-3) and sternocleidomastoid (SCM) (Fig. 25-3). Other superficial muscles, which connect the scapula with the vertebral column, include the levator scapulae and the rhomboids. The scalene muscle group (Fig. 25-4) provides a relationship between the cervical spine and the first and second ribs.
FIGURE 25-3 Muscles of the lateral and posterior cervical spine. (Reproduced, with permission, from Chapter 25. Overview of the Neck. In: Morton DA, Foreman K, Albertine KH. eds. The Big Picture: Gross Anatomy. New York, NY: McGraw-Hill; 2011.)
FIGURE 25-4 Muscles of the anterior cervical spine. (Reproduced, with permission, from Chapter 25. Overview of the Neck. In: Morton DA, Foreman K, Albertine KH. eds. The Big Picture: Gross Anatomy. New York, NY: McGraw-Hill; 2011.)
A deeper layer links the skull and vertebral column and includes long posterior (dorsal), splenius capitis (Fig. 25-5), semispinalis capitis, and longissimus capitis.
FIGURE 25-5 Deep cervical muscles. (Reproduced, with permission, from Chapter 1. Back. In: Morton DA, Foreman K, Albertine KH. eds. The Big Picture: Gross Anatomy. New York, NY: McGraw-Hill; 2011.)
The deepest layers consist of muscles that link the cervical and thoracic vertebrae, splenius cervicis, semispinalis cervicis, and longissimus cervicis.
For the most part, the muscles of the neck function to support and move the head. Given the number of degrees of freedom available at the neck, it is likely that the muscles are organized as functional synergies. Muscle synergies are conceptualized as units of control, incorporating the muscles around the joint that will act together in a functional fashion.52 Under this concept, the central nervous system (CNS) needs only trigger a synergic unit to produce a specific movement, instead of communicating with each individual muscle.52 Synergistic movements incorporate both agonist and antagonist muscle groups, which results in a greater level of control.
Superficial Muscles
Trapezius
The trapezius muscle (Fig. 25-3) is the most superficial back muscle. It is a flat triangular muscle that runs from the superior nuchal line and external occipital protuberance of the occipital bone to the spinous process of T12 and is the largest muscle attachment in the body. Its insertion can be traced from the entire superior aspect of the spine of the scapula, the medial aspect of the acromion, and the posterior aspect of the lateral third of the clavicle.
This muscle is traditionally divided into middle, upper, and lower parts, according to anatomy and function.
The middle part originates from C7 and forms the cervicothoracic part of the muscle.
The lower part, attaching to the apex of the scapular spine, is relatively thin.
The upper part (Table 25-1) is very thin, and yet it has the most mechanical and clinical importance to the cervical spine.53
TABLE 25-1 | Attachments of Upper Trapezius and Levator Scapulae Muscles |
Muscle | Proximal | Distal | Innervation |
Upper trapezius | Superior nuchal line Ligamentum nuchae | Lateral third of clavicle and the acromion process | Spinal accessory |
Levator scapulae | Transverse processes of upper four cervical vertebrae | Medial border of scapula at level of scapular superior angle | Posterior (dorsal) scapular C5 (C3 and C4) |
The innervation of the trapezius comes from the accessory nerve (CN XI) and fibers from the anterior (ventral) rami of the third and fourth cervical spinal nerves, with the former being speculated to provide the motor innervation and the latter supplying the sensory information.54–58 The greater occipital nerve occasionally travels through the trapezius near its superior border to reach the scalp and can become entrapped by an adaptive shortening of the upper trapezius muscle or be traumatized by a blunt force.58–60
The different parts of this muscle provide a variety of actions on the shoulder girdle, including elevation and retraction of the scapula. When the shoulder girdle is fixed, the trapezius can produce ipsilateral side bending and contralateral rotation of the head and neck. Working together, the trapezius muscles can produce symmetric extension of the neck and head.61 In addition, the trapezius muscle can produce scapular adduction (all three parts), and upward rotation of the scapula (primarily the superior and inferior parts). The importance of the trapezius to the shoulder joint is discussed in Chapter 16.
Sternocleidomastoid
The SCM (Fig. 25-3) is a fusiform muscle that descends obliquely across the side of the neck, forming a distinct landmark for palpatory purposes. It is the largest muscle in the anterior neck. It is attached inferiorly by two heads, arising from the posterior aspect of the medial third of the clavicle and the manubrium of the sternum. From here, it passes superiorly and posteriorly to attach to the mastoid process of the temporal bone. The motor supply for this muscle is from the accessory nerve (CN XI), whereas the sensory innervation is supplied by the anterior (ventral) rami of C2 and C3.62 This muscle can provide the clinician with information regarding the severity of symptoms, and postural impairments, because of its tendency to become prominent when hypertonic. The SCM is also involved with a condition called torticollis, a postural deformity of the neck (see Chapter 5 and later).
In broad terms, the actions of this muscle are flexion, side bending, and contralateral rotation of the head and neck.61 The two muscles act together to draw the head forward and can also raise the head when the body is supine. This head-raising action is a combination of upper cervical extension and lower cervical flexion. The muscle is also active on resisted neck flexion. With the head fixed, it is also an accessory muscle of forced inspiration.
Levator Scapulae
The levator scapulae (Fig. 16-5) is a slender muscle attached by tendinous slips to the posterior tubercles of the transverse processes of the upper cervical vertebrae (C1–4). The levator, located deep to both the upper and the middle parts of the trapezius, can be palpated just deep to the superior border of the trapezius. It descends posteriorly, inferiorly, and laterally to the superior angle and medial border of the scapula, between the superior angle and the base of the spine (Table 25-1). The levator is the major stabilizer and elevator of the superior angle of the scapula. With the scapula stabilized, the levator produces rotation and side bending of the neck to the same side; when acting bilaterally, cervical extension is produced. With a forward head posture, the potential for this extension moment increases.1 This abnormal anterior translation is resisted by tension within the levator scapulae and the ligamentum nuchae.63
The levator is supplied by direct branches of the C3 and C4 cervical spinal nerves and from C5 through the posterior (dorsal) scapular nerve. It is heavily innervated with muscle spindles.
Rhomboids
The rhomboid major is a quadrilateral sheet of muscle, and the rhomboid minor muscle is small and cylindrical (see Chapter 16). Together they form a thin sheet of muscle that fills much of the interval between the medial border of the scapula and the midline. Although the rhomboid minor, with its attachment to the spinous processes of C7 and T1, has a slight association with the cervical spine, the rhomboid major, arising from the spinous processes of T1–5, is inactive during isolated head and neck movements. The two muscles descend from their points of origin, passing laterally to the posterior aspect of the vertebral border of the scapula, from the base of the spine to the inferior angle. Both these muscles receive their nerve supply from the posterior (dorsal) scapular nerve (anterior [ventral] ramus of C4–5). The principal action of these muscles is to work with the levator scapulae to control the position and movement of the scapula. Both the muscles are involved with concentric contractions during rowing exercises or other activities involving scapular retraction.
Scalenes
The scalenes (Fig. 25-4) extend obliquely like ladders (scala means ladder in Latin) and share a critical relationship with the subclavian artery. Tightness of these muscles will affect the mobility of the upper cervical spine. In addition, because of their distal attachments to the first and second ribs (Table 25-2), they can, if in spasm, elevate the ribs and be implicated in thoracic outlet syndrome (TOS).64,65
TABLE 25-2 | Attachments of Scalene, Longus Colli, and Longus Capitis Muscles |
Muscle | Proximal | Distal | Innervation |
Scalenus | |||
Anterior | Anterior tubercles of C3–6 | Superior crest of first rib | Anterior (ventral) rami of C3-8 |
Middle | Posterior tubercles of C2–7 | Superior crest of first rib | Anterior (ventral) rami of C3-8 |
Posterior | Posterior tubercles of C5–7 | Outer surface of second rib | Anterior (ventral) rami of C3-8 |
Longus colli | Anterior tubercles of C3–5 Anterior surface of C5–7, T1–3 | Tubercle of the atlas, anterior tubercles of C5 and C6, and anterior surface of C2–4 | Anterior (ventral) primary rami of cervical spinal nerves |
Longus capitis | Anterior tubercles of C3–6 | Inferior occipital bone and basilar portion | Anterior (ventral) primary rami of cervical spine nerves |
Scalenus Anterior. The scalenus anterior (see Fig. 25-4) runs vertically, behind the SCM on the lateral aspect of the neck. Arising from the anterior tubercles of the C3–6 transverse processes, it travels to the scalene tubercle on the inner border of the first rib. The osteal portion of the vertebral artery and the stellate ganglion are located lateral to the scalenus anterior. Acting from above, the scalenus anterior, like the rest of the scalenes, is an inspiratory muscle, even with quiet breathing.66 Working bilaterally from below, it flexes the spine. Unilaterally, it side bends the spine ipsilaterally and rotates the spine contralaterally. It is supplied by the anterior (ventral) rami of C3-8.
Scalenus Medius. The scalenus medius (see Fig. 25-4) is the largest and longest of the group, attaching to the transverse processes of all cervical vertebrae except the atlas (although it often attaches to this) and running to the upper border of the first rib. It is separated from the anterior scalene by the carotid artery, and cervical nerve, and is pierced by the nerve to the rhomboids (posterior [dorsal] scapular) and the upper two roots of the nerve to the serratus anterior (long thoracic). When working unilaterally on the cervical spine, the scalenus medius is an ipsilateral side bender of the neck. When working bilaterally, it is a cervical flexor.
Scalenus Posterior. The scalenus posterior (Fig. 25-4) is the smallest and deepest of the group, running from the posterior tubercles of the C4–6 transverse processes to attach to the outer aspect of the second rib. It functions to elevate or fix the second rib and side bends the neck ipsilaterally. It is innervated by the anterior (ventral) rami of C3-8.
Scalenus Minimus (Pleuralis). The scalenus minimus is a small muscle slip, running from the transverse process of C7 to the inner aspect of the first rib and the dome of the pleura. It is a suprapleural membrane that is often considered to be the expansion of the tendon of this muscle. The muscle functions to elevate the dome of the pleura during inspiration and is innervated by the anterior (ventral) ramus of C7.
Platysma. The broad sheet of the platysma muscle is the most superficial muscle in the cervical region (Fig. 25-3). The platysma covers most of the anterolateral aspect of the neck, the upper parts of the pectoralis major, and deltoid, extending superiorly to the inferior margin of the body of the mandible. As a muscle of facial expression, it cannot affect bony motion, except perhaps as a passive restraint to head extension. It is supplied by the cervical branch of CN VII (facial).
Deeper Muscles of the Back
The deep or intrinsic muscles of the back are the primary movers of the vertebral column and head and are located deep to the thoracolumbar fascia. The muscles in all of these groups are segmentally innervated by the lateral branches of the posterior (dorsal) rami of the spinal nerves.
Splenius Capitis
The splenius capitis (Fig. 25-5) extends upward and laterally, from the posterior (dorsal) edge of the nuchal ligament and the spinous processes of the lower cervical and upper thoracic vertebrae (T4–C7) to the mastoid process of the occipital bone just inferior to the superior nuchal line and deep to the SCM muscle.
Splenius Cervicis
The splenius cervicis is just inferior and appears continuous with the capitis, extending from the spines of the third to the sixth thoracic vertebrae to the posterior tubercles of the transverse processes of the upper cervical vertebrae.
The splenius capitis and splenius cervicis muscles are two important head and neck rotators. From their attachments (Table 25-3), it is clear that these two muscles are capable of producing ipsilateral rotation, side bending, and extension at the spinal joints they cross.
TABLE 25-3 | Attachments of Splenius Capitis and Cervicis Muscles |
Muscle | Proximal | Distal | Innervation |
Splenius capitis | Inferior ligamentum nuchae, spinous process of C7 and T1–4 vertebrae | Mastoid process, occipital bone, and lateral third of superior nuchal line | Cervical spinal nerve and posterior (dorsal) primary rami of C 3-4 |
Splenius cervicis | Spinous processes of T3–6 vertebrae | Posterior tubercles of C1–3 | Posterior (dorsal) primary rami of C5, 6 |
Cervical Erector Spinae
The erector spinae complex spans multiple segments, forming a large musculotendinous mass (Fig. 25-6) consisting of the iliocostalis, longissimus, and spinalis muscles (Tables 25-4 and 25-5).
FIGURE 25-6 Erector spinae. (Reproduced, with permission, from Chapter 1. Back. In: Morton DA, Foreman K, Albertine KH. eds. The Big Picture: Gross Anatomy. New York, NY: McGraw-Hill; 2011.)
TABLE 25-4 | Prime Movers of the Cervical Spine: Rotation and Side Bending |
Muscles of Rotation and Side Bending | |
Ipsilateral side bending | Ipsilateral rotation |
Longissimus capitis | Splenius capitis |
Intertransversarii posteriores cervices | Splenius cervicis |
Multifidus | Rotatores breves cervicis |
Rectus capitis lateralis | Rotatores longi cervicis |
Intertransversarii anteriores cervices | Rectus capitis posterior major |
Scaleni | Obliquus capitis inferior |
Iliocostalis cervicis | |
Contralateral rotation Obliquus capitis superior | Ipsilateral side bending and contralateral rotation Sternocleidomastoid Scalenus anterior Multifidus Longus colli |
Ipsilateral side bending and ipsilateral rotation Longus coli Scalenus posterior | |
Data from Evjenth O, Hamberg J. Muscle Stretching in Manual Therapy, a Clinical Manual. Alfta, Sweden: Alfta Rehab Forlag; 1984. |
TABLE 25-5 | Prime Movers of the Cervical Spine: Extensors and Flexors |
Extensor Muscles | Flexor Muscles | |
Prime movers | Accessory muscles | Prime movers |
Trapezius | Multifidus | Sternocleidomastoid—anterior fibers |
Sternocleidomastoid—posterior fibers | Suboccipitals | Accessory muscles |
Iliocostalis cervicis | Rectus capitis posterior major and minor | Prevertebral muscles |
Longissimus cervicis | Obliquus capitis superior | Longus colli |
Splenius cervicis | Obliquus capitis inferior | Longus capitis |
Splenius capitis | Rectus capitis anterior | |
Interspinales cervicis | Scalene group | |
Spinalis cervicis | Scalenus anterior | |
Spinalis capitis | Infrahyoid group | |
Semispinalis cervicis | Sternohyoid | |
Semispinalis capitis | Omohyoid | |
Levator scapulae | Sternothyroid Thyrohyoid | |
Data from Evjenth O, Hamberg J. Muscle Stretching in Manual Therapy, a Clinical Manual. Alfta, Sweden: Alfta Rehab Forlag; 1984. |
The iliocostalis cervicis appears to function as a stabilizer of the cervicothoracic junction and lower cervical spine.
The semispinalis has thoracis, cervicis, and capitis divisions. The obliquus capitis superior (superior oblique) and inferior (inferior oblique) and the rectus capitis posterior major and minor (see Chapter 23) lie underneath the semispinalis capitis and splenius capitis muscles.67 The semispinalis cervicis is a stout muscle that extends superiorly to the spinous process of vertebra C2, functioning as a strong extensor of the lower cervical spine.1
The interspinales and intertransversarii, which interconnect the processes for which they are named, produce only minimal motion because they can influence only one motion segment and are more likely to function as sensory organs for reflexes and proprioception.68
Deep Neck Flexors
The prevertebral muscles of the neck consist of:
Longus colli. The longus colli (Fig. 25-4) consists of a vertical portion that originates from the bodies of the first three thoracic and last three cervical vertebrae, an inferior oblique portion that originates from the bodies of the first three thoracic vertebrae, and the superior oblique portion that originates from anterior tubercles of the transverse processes of C3–5. The various portions of the longus colli insert into the bodies of C2–4, the anterior tubercles of the transverse processes of C5–6, and the anterior tubercle of the atlas. The longus colli functions to flex (and assists in rotating) the cervical vertebrae and head. Acting singly, the longus colli side bends the vertebral column. The longus colli is innervated by branches of the anterior primary rami of C2–8.
Longus capitis. The longus capitis (Fig. 25-4) originates from the anterior tubercles of the transverse processes of C3–6 and inserts onto the inferior surface of the basilar part of the occipital bone. The longus capitis functions to flex (and assists in rotating) the cervical vertebrae and head. It is innervated by the muscular branches of C1–4.
Rectus capitis anterior. The rectus capitis anterior (RCA) originates from the lateral mass of the atlas and inserts onto the base of the occipital bone anterior to the foramen magnum. The RCA flexes and rotates the head and is innervated by the muscular branches of C1–2.
Rectus capitis lateralis. The rectus capitis lateralis (RCL) originates from the upper surface of the transverse process of the atlas and inserts onto the inferior surface of the jugular process of the occipital bone. The RCL side bends the head to the ipsilateral side and is innervated by branches of the anterior rami of C1–2.
Neurology
The cervical spine is the only region that has more nerve roots than vertebral levels.8
In general, structures supplied by the upper three cervical nerves can cause neck and head pain (see Table 23-1), whereas the mid to lower cervical nerves can refer symptoms to the shoulder, anterior chest, upper limb, and scapular area.69
Cervical proprioceptive input has considerable influence on posture through the tonic neck reflex and on eye movement and accommodation through the cervico-occular and vestibulo-ocular reflexes (see Chapter 3).69–71 It is probably no accident that the two largest postural muscles of the head and neck, the trapezius, and the SCM muscles, are partly innervated by the accessory nerve (CN XI).
Vascular Supply
The middle cervical segments are supplied by radicular branches off the extradural vertebral artery and are the most common segments to be affected in vertebral artery disease.72 The vertebral artery is detailed in Chapter 24.
The common carotid artery bifurcates at the middle to upper cervical level into the internal and external carotid arteries. The carotid body, a specialized structure that senses oxygen and carbon dioxide levels in the blood, is located at this bifurcation.10 The carotid sinus, which contains baroreceptors that monitor blood pressure, is located at a point before the bifurcation.10
Cervical Lordosis
The amount of cervical lordosis is a factor of the zygapophyseal joint planes and the cervical IVDs. Under normal conditions, the C4–5 interspace is considered the midpoint of the curve, and the center of gravity (COG) for the skull lies anterior to the foramen magnum. The longus colli has been shown to have an important supporting role on the cervical curve.73
A reduction in the cervical lordotic curve because of injury or abnormal posture results in more weight being borne by the vertebral bodies and IVDs, whereas an increase in the lordosis increases the compressive load on the zygapophyseal joints and posterior elements. Watson and Trout74 have demonstrated a link between a lack of endurance capacity of the upper cervical and deep neck flexors and the forward head posture (see later).
Cervicothoracic Junction
The cervicothoracic junction comprises the C7–T1 segment, although functionally it includes the seventh cervical vertebra, the first two thoracic vertebrae, the first and second ribs, and the manubrium. In addition, the cervicothoracic junction forms the thoracic outlet, through which the neurovascular structures of the upper extremities pass. Lewit75 considers the cervicothoracic junction to be the third major area of the spine for musculoskeletal problems, with the craniovertebral area and the lumbosacral junction being first and second, respectively.
BIOMECHANICS
The motions that occur in the cervical spine correspond to motions of the head, although the range of head movement bears little relation to the range of neck movement and that the total range is the sum of both the head and the neck motions.76
The cervical spine is a complex mechanical linkage composed of multiple degrees of freedom of movement about each of its joints and at least 20 pairs of muscles, many of which are capable of performing similar actions.52 It is estimated that the osseoligamentous system contributes 20% to the mechanical stability of the cervical spine while 80% is provided by the surrounding neck musculature.77 The role of the ligaments in stabilization occurs mainly at the end-of-range postures,78 while the muscles supply dynamic support in activities around neutral- and midrange postures.79
At the zygapophyseal joints, there is a sagittal range of 50–80 degrees in each direction of flexion and extension.80 The only significant arthrokinematic motions available to the zygapophyseal joint are an inferior, medial glide of the inferior articular process of the superior facet during extension and a superior, lateral glide during flexion. Segmental side bending is, therefore, an extension of the ipsilateral joint and flexion of the contralateral joint. Rotation, coupled with ipsilateral side bending, also involves the extension of the ipsilateral joint and flexion of the contralateral. Because of their multiple insertions, many of the neck muscles have multiple functions or can change their function depending on the initial position of each vertebral joint and the degree to which the joints are free to move in each of the planes of motion.52 Thus, a voluntary motor task of the head and neck can be accomplished through a variety of combinations of kinematic and muscle actions. Considering that most functional daily tasks are performed in and around midrange postures, the muscles controlling the cervical spine are subject to constant external and internal forces.79
Flexion
Flexion may be divided into three sequential phases.81,82 The initial phase begins in the lower cervical spine (C4–7) where C6–7 makes its maximum contribution followed by the C5–6 segment and then by C4–5. Motion in the second phase occurs initially at C0–2, followed by C2–3 and C3–4. The first phase of motion occurs again at the lower cervical spine (C4–7) initially, with the C4–5 segment followed by C5–6 then the C6–7 segments.81,82
At the segmental level, flexion is described as an anterior osteokinematic rock tilt of the superior vertebra in the sagittal plane, a superoanterior glide of both superior facets of the zygapophyseal joints, and an anterior translation slide of the superior vertebra on the IVD (see Fig. 25-7). This produces an anterior (ventral) compression and a posterior (dorsal) distraction of the cervical disk. The uncovertebral joint lies on, or very near to, the axis of rotation of flexion and extension. Consequently, the main arthrokinematic motion that seems likely to be occurring here is an anterior spin (or very near spin).83 This arthrokinematic spin appears especially probable, as impairments of the uncovertebral joint seem to be unaffected by flexion or extension. Thus, uncovertebral restrictions may be detected in all cervical positions, although flexion partly disengages the joint because of its posterior position on the vertebra.83
FIGURE 25-7 Kinematics of craniocervical flexion.
Although all the following anatomic movement restrictors act to some degree on most of the components of flexion, they act particularly on the associated movement component.
Anterior osteokinematic motion is restrained by the extensor muscles and the posterior ligaments (posterior longitudinal, interspinous, and ligamentum flavum).
Superoanterior arthrokinematic is restrained by the joint capsule, whereas translation is restrained by the disk and the nuchal ligament.
Extension
Extension is described as a posterior osteokinematic sagittal rock, an inferoposterior glide and approximation of the superior facets of the zygapophyseal joints, and a posterior translation of the vertebra on the disk (see Fig. 25-8). The uncovertebral joint undergoes a posterior arthrokinematic spin. The osteokinematic motion of extension is restricted by the anterior prevertebral muscles and the ALL. The arthrokinematic motion is restricted by the zygapophyseal joint capsule.83 The IVD restrains the posterior translation.
FIGURE 25-8 Kinematics of craniocervical extension.
Side Bending
Side bending (lateral flexion) is an ipsilateral osteokinematic rock, a superoanterior glide of the contralateral superior facet, and a posteroinferior glide of the ipsilateral facet (see Fig. 25-9). In addition, there is a contralateral translation of the vertebra on the disk, an inferomedial glide of the ipsilateral uncovertebral joint, and a superolateral glide of the contralateral uncovertebral joint. A composite curved translation results. This curve is formed by the superoinferior linear glides of the zygapophyseal joints, the oblique inferomedial and superomedial glides of the uncovertebral joints, and the linear translation across the disk.83
FIGURE 25-9 Kinematics of craniocervical side bending.
The osteokinematic rock can be limited by the contralateral scalenes and intertransverse ligaments. The uncovertebral and zygapophyseal arthrokinematic motions can be limited by the joint capsule, and the translation is limited by the IVD. If the side bending is limited, but the translation is okay, it is unlikely that the joint complex (the zygapophyseal joint, disk, or uncovertebral joint) is impaired; instead, these findings may implicate adaptive shortening of the soft tissues.83 However, if the translation is also limited, a problem with the joint complex also probably exists.
Rotation
Rotation is chiefly an osteokinematic motion of the vertebra about a vertical axis that is coupled with ipsilateral side bending (see Fig. 25-10). Presumably, the translation follows the side bending (i.e., contralateral), resulting in the same uncovertebral and zygapophyseal arthrokinematic motions as does side bending.83
FIGURE 25-10 Kinematics of craniocervical rotation.
Muscle Control
The control of head and neck postures and movements is a complex task, especially in the presence of pain or dysfunction. The muscle groups of the cervical region may be divided into those that sustain postures or stabilize the segments and those that produce movement.84–86 Considerable biomechanical, physiological, and clinical research in both the cervical and lumbar regions has been undertaken which supports Bergmark’s86 model for a functional division between the deep and more superficial muscles in their relative contributions to spinal support and control.79 According to this model, the more superficial multisegmental (global) muscles have the responsibility for maintaining equilibrium of external forces (torque production and control of the head) so that the load transmitted to the spinal segments can be controlled efficiently by the deep intersegmental (local) muscle system.69,79,86
The SCM (anteriorly) and the semispinalis capitis and splenius capitis (posteriorly) are thought to be the global muscles of the neck, whereas the local system is thought to comprise the longus capitis and colli (Table 25-2),73 semispinalis cervicis, and multifidus.87 The longus colli and posterior (dorsal) neck muscles form a muscle sleeve that surrounds the cervical column to support the cervical spinal segments in functional movements.73
Patients with neck pain have been found to demonstrate generally less torque production (strength and endurance) in all planes, with a greater loss in the cervical flexors than extensors.88 However, addressing strength alone in research and exercise programs may oversimplify the problems in the neuromuscular system associated with neck pain.79 Recent research has recognized the vital role that activation of the deep neck flexor muscles plays in supporting the cervical segments and the cervical curve (see “Muscle Function Testing: Deep Neck Flexors” section later).73,87,89 Indeed, impairment in the axioscapular-girdle muscles has been linked clinically to cervical pain syndromes.84,90–94 The nature of the impairment in the cervical and cervicobrachial muscle systems indicates the need for careful and precise movement analysis and muscle testing to appreciate and accurately define the problem.79
EXAMINATION
The cervical spine is an area with a high potential for serious injury, which makes this a region of the body that needs to be examined with caution, especially when there is a history of acute and recent trauma of the neck, because of the potential for the examination itself to be harmful.95 Although most conditions involving the neck and upper limb symptoms can be diagnosed after a careful history and physical examination, in cases of significant trauma, imaging studies may be required to exclude a fracture or instability. Where possible, the patient should be examined for central and/or peripheral neurologic deficit, neurovascular compromise, and serious skeletal injuries, such as fractures or craniovertebral ligamentous instability.
Winkel et al.96 clinically categorize cervical disorders by the location of symptoms and the etiology of each condition:
Local cervical syndrome (LCS). This syndrome manifests with local neck complaints, resulting from cervical muscle strain, ligament sprain, or a primary or secondary disk-related condition.
A primary disk-related LCS is characterized by symptoms that may result from a protrusion, prolapse, or extrusion of the disk.
A secondary disk-related LCS is characterized by symptoms resulting from gradual changes in the cervical spine that have been generated by the previous degradation of the IVD. These changes include internal disk disruption and synovitis or irritation of the zygapophyseal and uncovertebral joints. In the cervical spine, myofascial pain may be a secondary tissue response to an IVD or zygapophyseal joint injury.97 The cervical zygapophyseal (facet) joints can be responsible for a significant portion of chronic neck pain. Established referral zones for the cervical zygapophyseal joint98,99 overlap both myofascial and dermatomal pain patterns. Cervical zygapophyseal joint pain is typically unilateral and is described by the patient as a dull ache. Occasionally, the pain can be referred to the craniovertebral or interscapular regions.
Cervicobrachial syndrome. This syndrome includes symptoms in the local cervical region, as well as in one or both upper extremities, as a result of nerve root irritation by compression or tension of the nerve root.
Cervicocephalic syndrome. This syndrome is characterized by complaints in both the neck and the head and includes symptoms such as dizziness, tinnitus, and headache. These symptoms may result from articular, ligamentous, neurologic, organic, or vascular sources.
Cervicomedullary syndrome. This syndrome is characterized by spinal cord symptoms associated with cord compression at the cervical spine (cervical myelopathy).

Stay updated, free articles. Join our Telegram channel

Full access? Get Clinical Tree

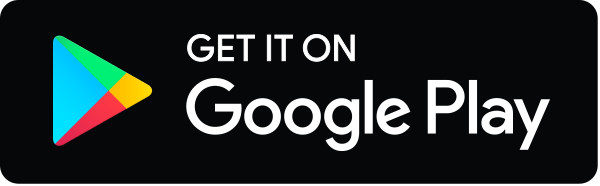
